As a winter storm moved through the Northeast U.S. the weekend of Jan. 21-22, some 40 million residents watched the forecasts, wondering who would get the rain, who would get the snow, and who would get the “wintry mix.”
This Nor’easter was a tricky one to forecast. Temperatures near the coast were expected to be near (or above) freezing. Temperatures inland were expected to be much colder. “Liquid-equivalent precipitation,” according to the Global Forecast System, was predicted in the 1-3 inch (25-75 mm) range. (Liquid-equivalent precipitation is the amount that would fall if it were liquid, though it is often sleet or snow.) This could easily convert to 1-2 feet (30-60 cm) of snow. It was the kind of situation that meteorologists live for.
While the difference between 71 and 74 degrees Fahrenheit is virtually meaningless, the difference between 31 and 34 degrees Fahrenheit (with heavy precipitation, at least) is the difference between closing schools, bringing out the plows, and shutting down public transportation—or life as usual.
Of course, the obvious follow-up question is what will the “wintry mix” be? Rain mixed with snow? Sleet? Freezing rain? It doesn’t take much to change from one to the other, but what ultimately falls from the sky can make a big difference to the people below.
So in the case of this storm, what happened? Here’s an article that explains it well. And here are PDF files of the storm reports from National Weather Service forecast offices in Albany, Norton, Mass., and Upton, N.Y. The synopsis: some places received 1.5 inches (~38 mm) of rain, some places received 11 inches (30 cm) of snow, and some places were coated in up to 0.6 inches (15 mm) of ice.
Consider the locations that received the ice. Plotted on a map, areas with more than 0.1 inches of ice match up quite well with storm-related power outages. Scroll down in this article to see a map of outages in Connecticut during this time period.
Now, compare that map with this NOAA-20 VIIRS image from January 22 (captured after the clouds cleared):
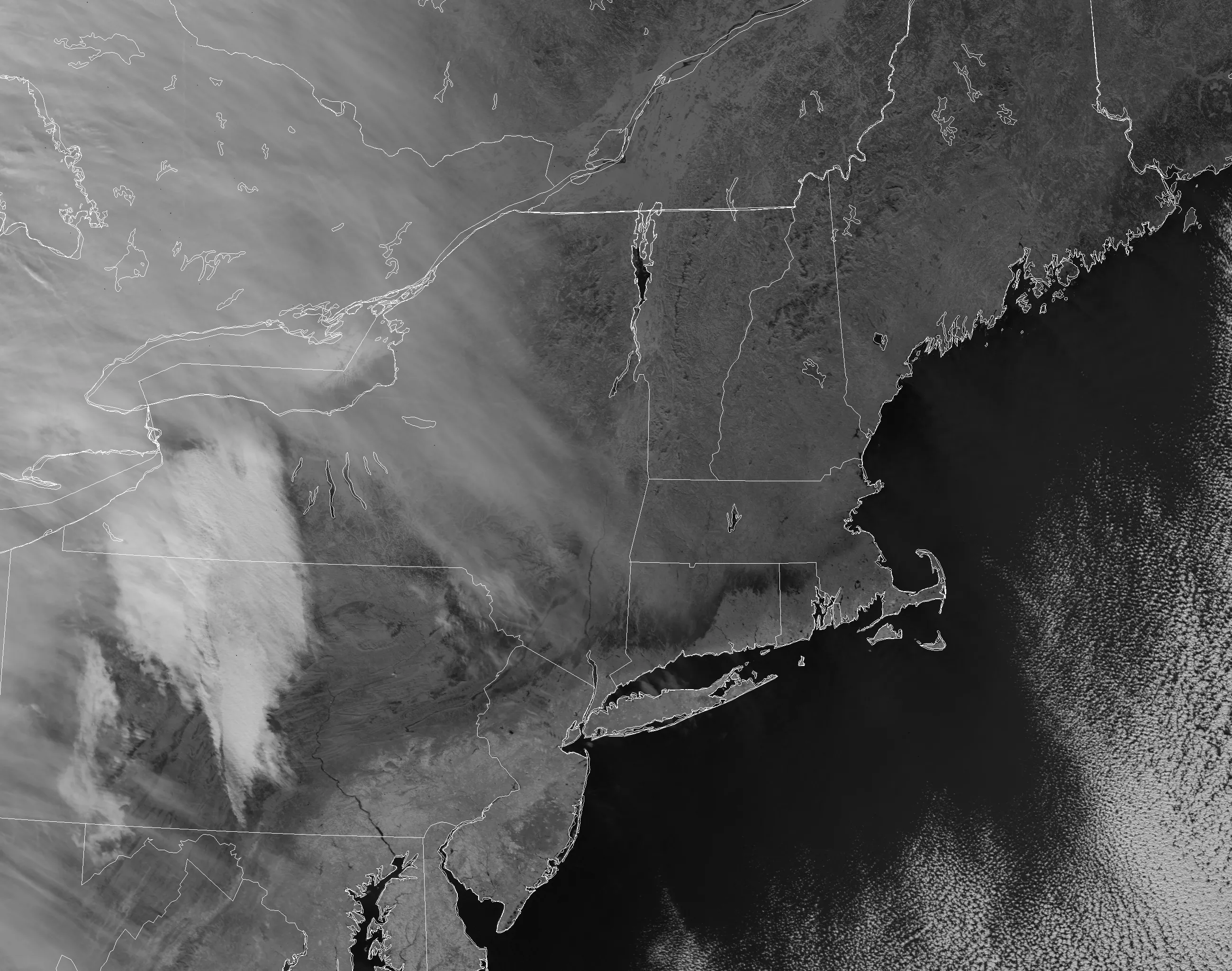
VIIRS channel I-3 image from NOAA-20, 17:09 UTC 22 January 2019. Image courtesy Curtis Seaman (CIRA/Colorado State University)
You can click on the image to bring up the full resolution version. This is the high-resolution imagery band, I-3, centered at 1.6 µm from the NOAA-20 satellite. Notice that very dark band stretching from northern New Jersey into northern Rhode Island? That is the area of greatest ice accumulation. And notice how well it corresponds with the known power outages across Connecticut!
The ice-covered region appears dark at 1.6 µm because ice is very absorbing at this wavelength and, hence, not very reflective. And, since it is cold, it doesn’t emit radiation at this wavelength either (at least, not in any significant amount). This is especially true for pure ice, as was observed here (particularly in the second image), since there aren’t any impurities in the ice to reflect radiation back to the satellite. The absorbing nature of snow and ice compared with the reflective nature of liquid clouds is what earned this channel the nickname “Snow/Ice Band” (PDF).
At shorter wavelengths (less than ~ 1 µm), ice and snow are reflective. (Note how a coating of ice makes everything sparkle in the sunlight.) This makes it nearly impossible to identify ice accumulation in True Color images:
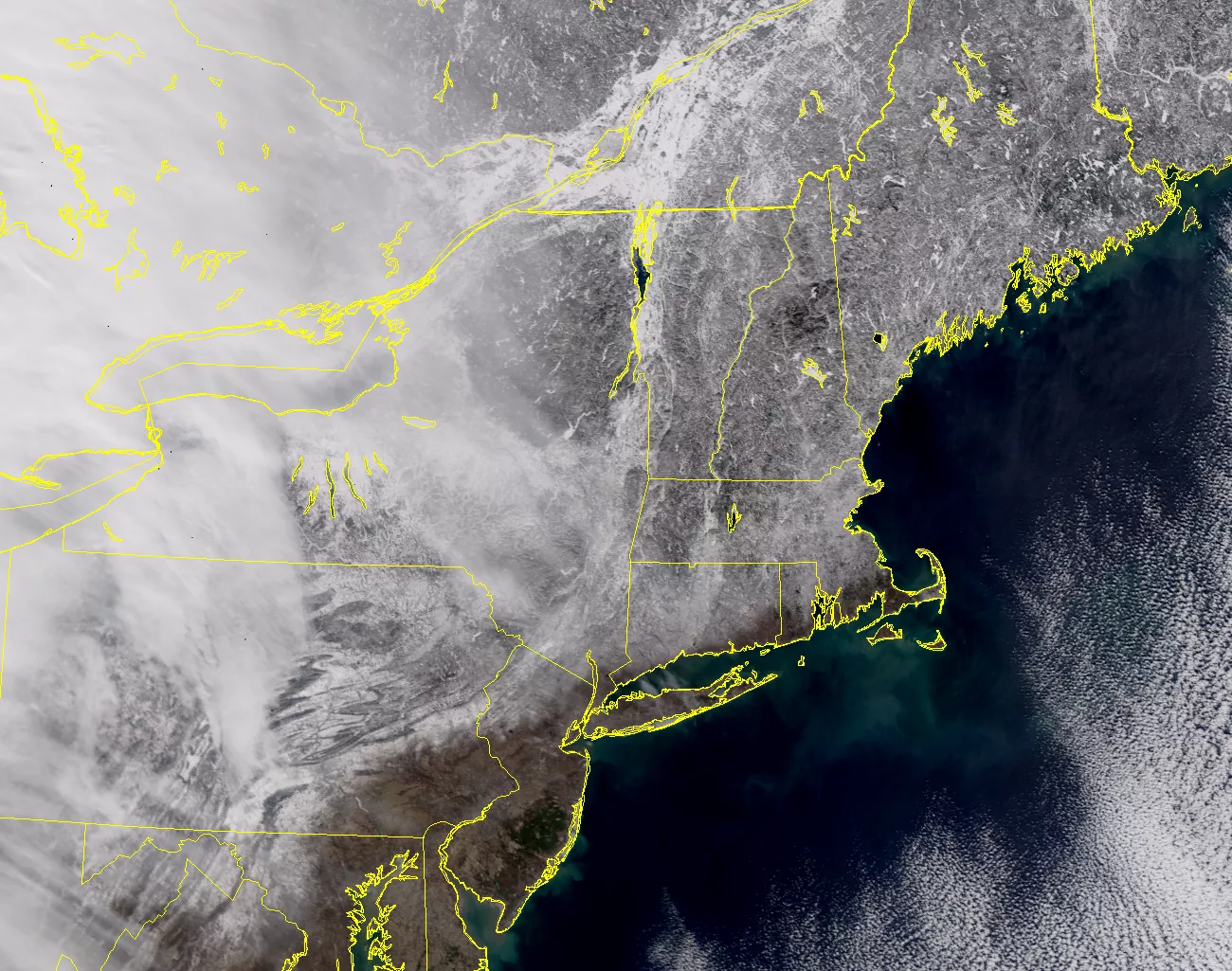
VIIRS True Color RGB composite of channels M-3, M-4 and M-5 from NOAA-20, 17:09 UTC 22 January 2019. Image courtesy Curtis Seaman (CIRA/Colorado State University)
The Natural Color RGB (which the National Weather Service forecasters know as the Day Land Cloud RGB) includes the 1.6 µm band, which is useful for distinguishing clouds from snow and ice. And, as expected, the region of ice accumulation does show up here (although it is tempered by the highly reflective nature of snow and ice in the visible and “veggie” bands that make up the other components of the RGB):
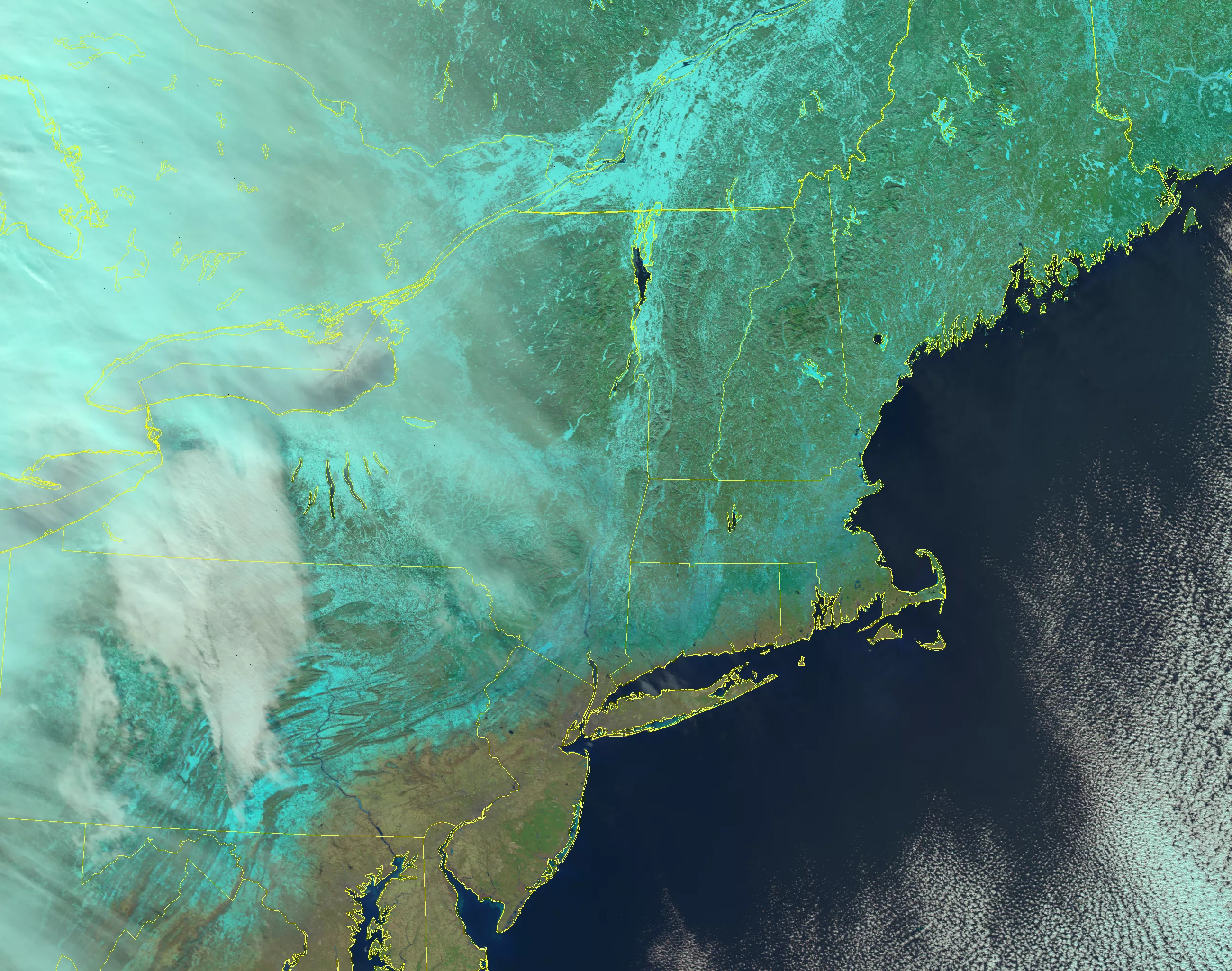
VIIRS Natural Color RGB composite of channels, I-1, I-2 and I-3 from NOAA-20 (17:09 UTC, 22 January 2019) Image courtesy Curtis Seaman (CIRA/Colorado State University)
Another RGB composite popular with forecasters is the Day Snow/Fog RGB, where blue is related to the brightness temperature difference between 10.7 µm and 3.9 µm, green is the 1.6 µm reflectance, and red is the reflectance at 0.86 µm (the “veggie” band). This shows the region of ice even more clearly than the Natural Color RGB:
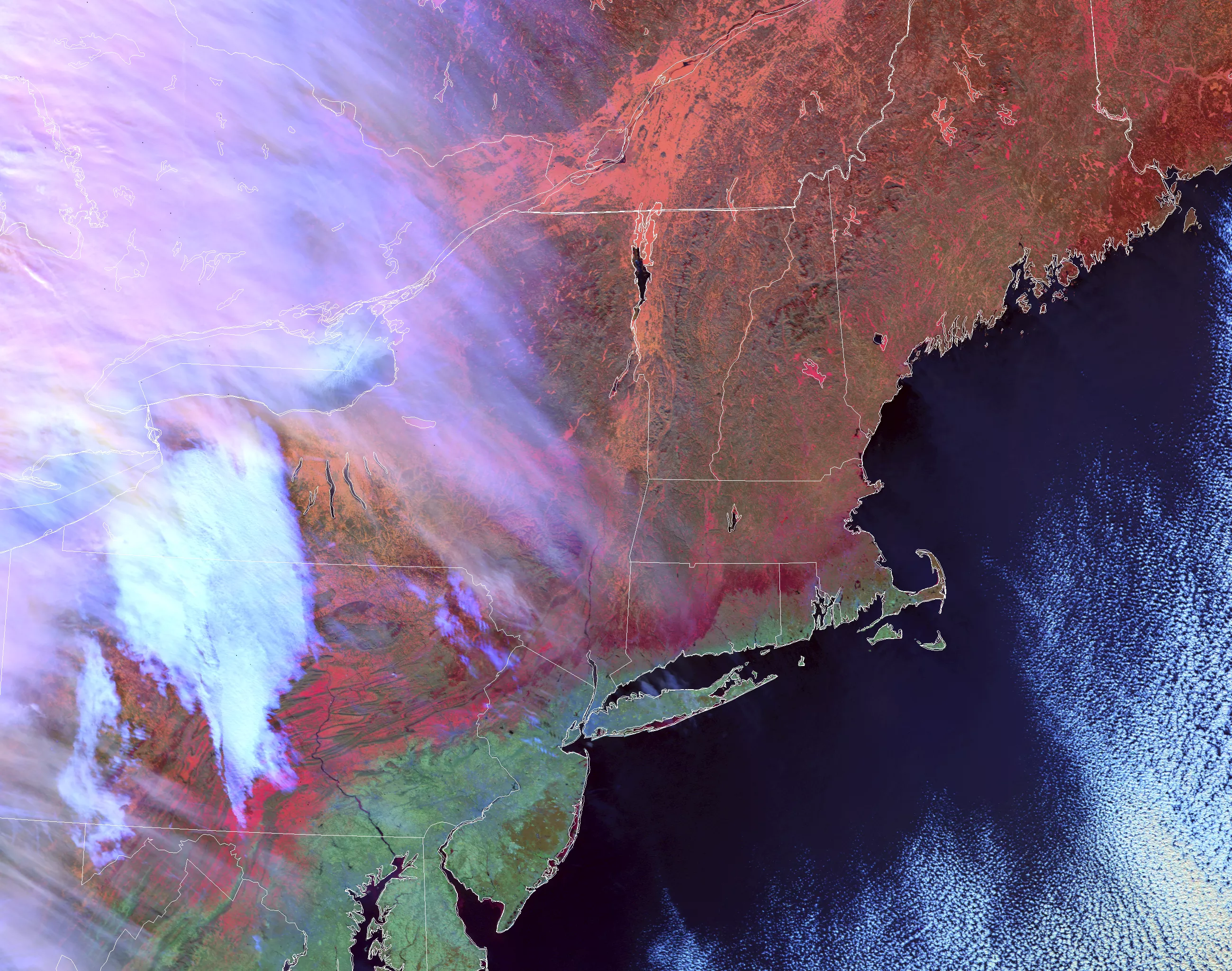
VIIRS Day Snow/Fog RGB composite of channels (I-5 minus I-4), I-3 and I-2 from NOAA-20 (17:09 UTC, 22 January 2019). Image courtesy Curtis Seaman (CIRA/Colorado State University)
You can see how the ice transitions from being reflective in the visible and near-infrared (near-IR) to absorbing in the shortwave-IR:
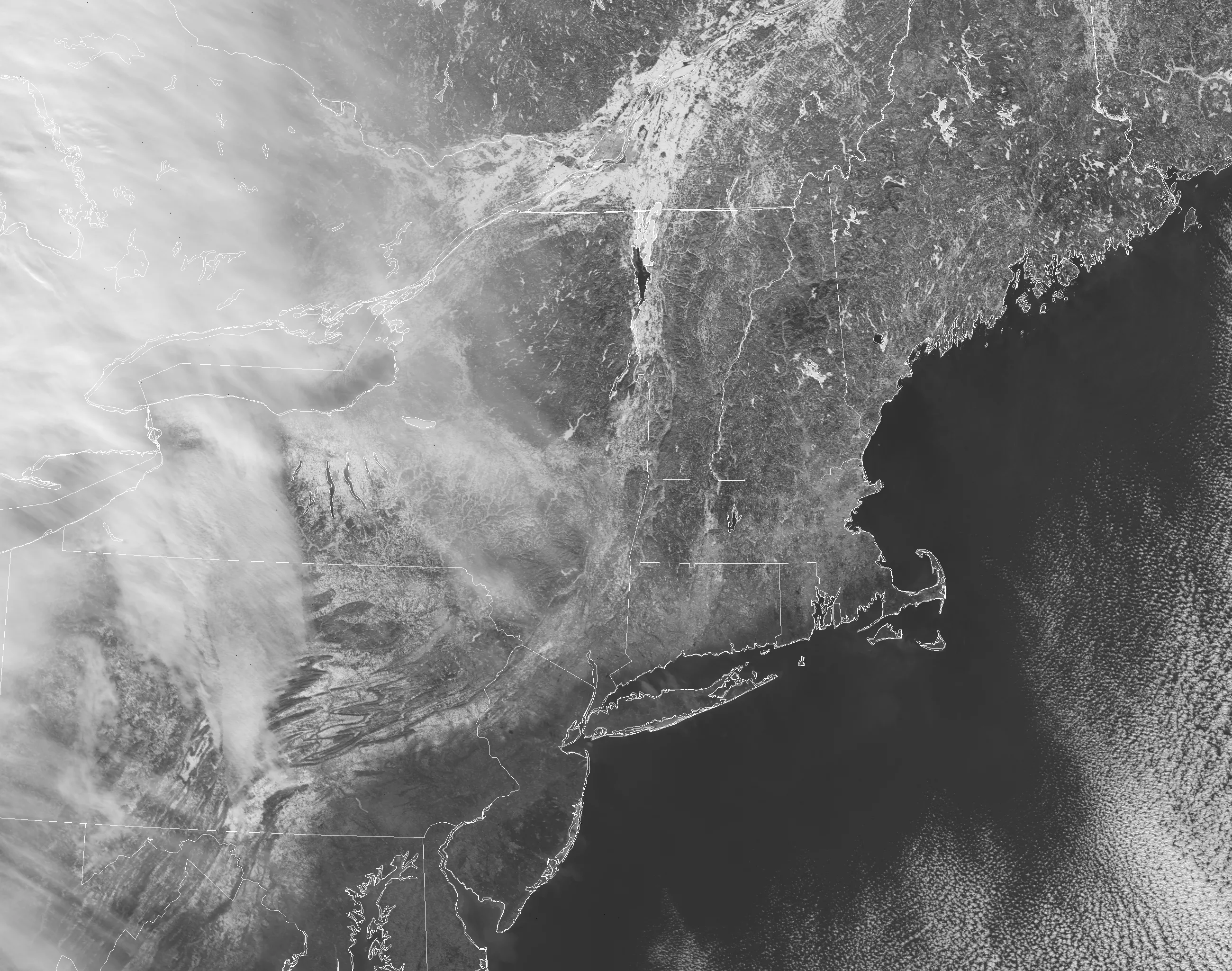
VIIRS high-resolution visible channel, I-1 (0.64 µm), from NOAA-20 (17:09 UTC, 22 January 2019). Image courtesy Curtis Seaman (CIRA/Colorado State University)
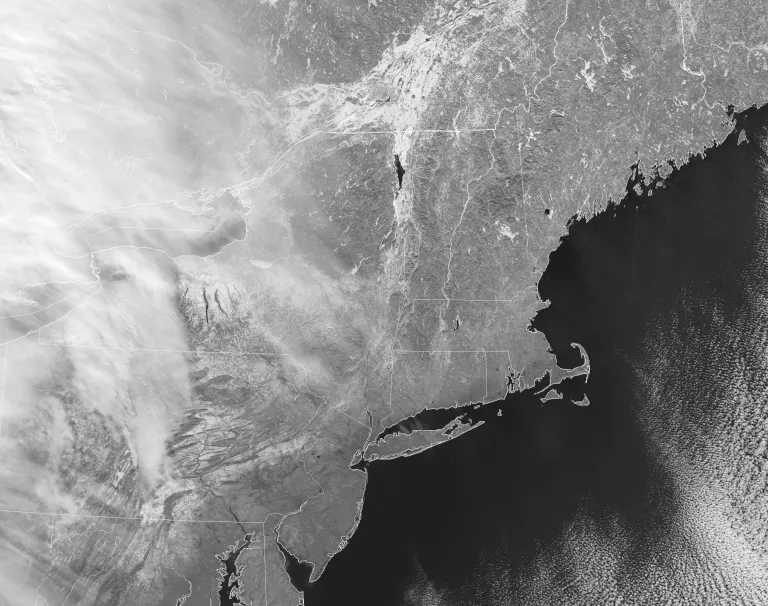
VIIRS high-resolution “veggie” channel, I-2 (0.86 µm), from NOAA-20 (17:09 UTC, 22 January 2019). Image courtesy Curtis Seaman (CIRA/Colorado State University)
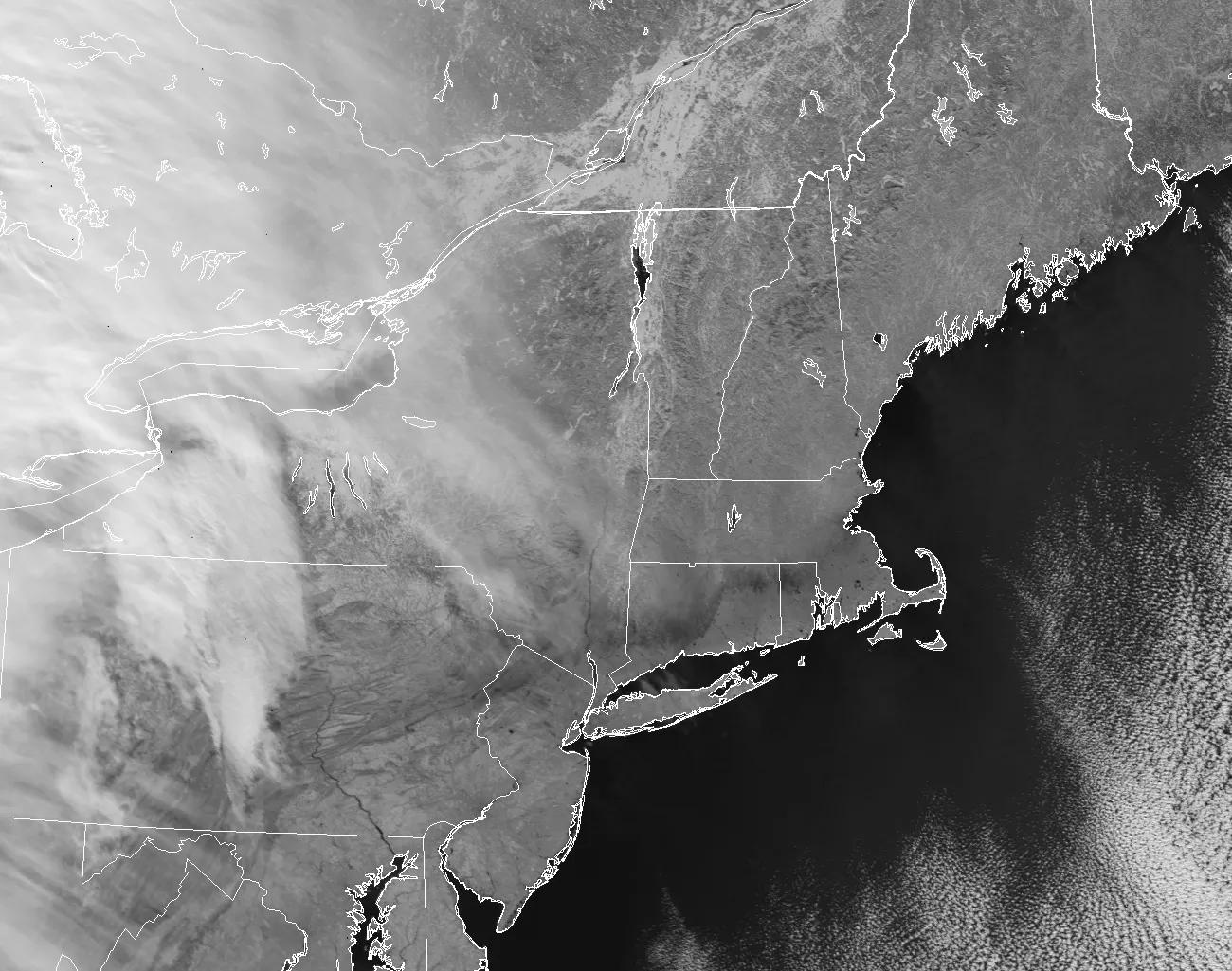
VIIRS channel M-8 (1.24 µm) from NOAA-20 (17:09 UTC, 22 January 2019). Image courtesy Curtis Seaman (CIRA/Colorado State University)
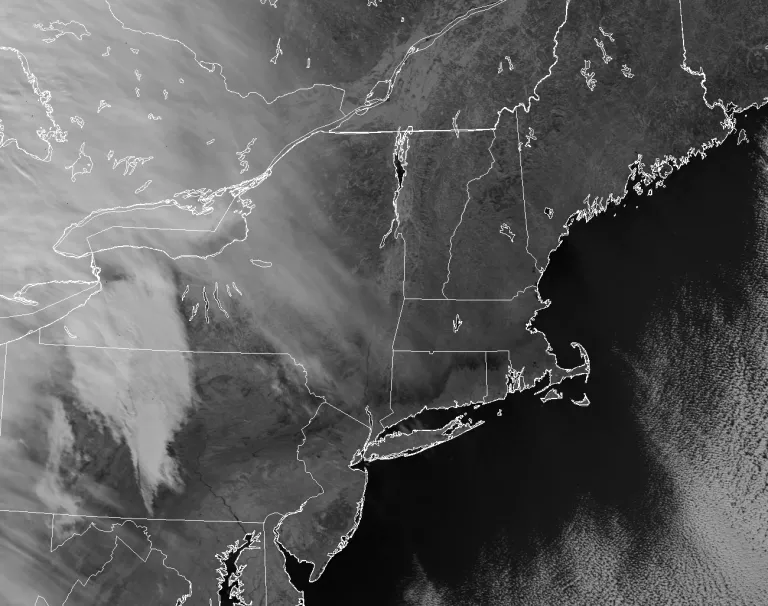
VIIRS channel M-11 (2.25 µm) from NOAA-20 (17:09 UTC, 22 January 2019). Image courtesy Curtis Seaman (CIRA/Colorado State University)
If you squint, you can even see a hint of the ice signature at 1.38 µm, the "Cirrus Band,” where most of the surface signal is blocked by water vapor absorption in the atmosphere:
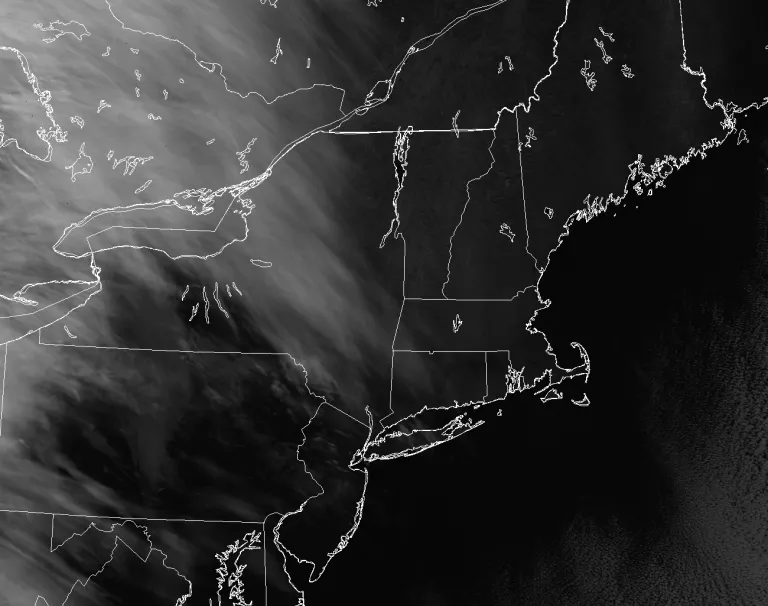
VIIRS “cirrus” channel, M-9 (1.38 µm), from NOAA-20 (17:09 UTC 22 January 2019). Image courtesy Curtis Seaman (CIRA/Colorado State University)
If the ice had accumulated in southern New Jersey or Pennsylvania, it would not have shown up in this channel, since the moisture in the air would have blocked the view of the surface. But you can see in this image why they call it the cirrus band.
So mark this down as another use for VIIRS: detecting areas impacted by ice storms. And remember, even though ice storms may have a certain beauty, they are dangerous. And, not just for the obvious reasons. During this storm, drivers in Connecticut reported a large number of “ice missiles,” chunks of snow and ice flying from the tops of vehicles. So, for the love of everyone else on the road, scrape your car clean of ice before attempting to drive.